Rosalba Bonaccorsi

Research Scientist
Disciplines: Astrobiology, Environmental Science, Marine Geochemistry, Biosedimentology
Degree/Major: Ph.D, Geological, Marine, and Environmental Sciences, 2001, University of Trieste, Italy
Curriculum vitae: 2024-10-9_BonaccorsiCV.pdf
rosalba.bonaccorsi-1@nasa.govDr. Rosalba Bonaccorsi is a Research Scientist at the SETI Institute and NASA Ames Research Center where she conducted Post-Doctoral research centered on source-to-sink of organics in a deep subsurface biosphere in Spain (2005-2008). In 2001 Rosalba obtained her Ph.D. in Geological, Marine, and Environmental Sciences at the University of Trieste (Italy). Formerly a teacher, she is an astrobiologist and an educator working with non-profit international, educational, and scientific organizations since 1989. For the past 16 years she has joined many NASA Spaceward Bound expeditions to extreme deserts worldwide (e.g., Atacama, Australia, Namibia, Mojave) were she served as Science Team member and Field Instructor for pre-service and in-service teachers enrolled in the expeditions. Rosalba joined the SETI Institute in 2009, and is keen to achieve a wide picture of where life and its signatures are most distributed, concentrated, preserved, and detected. Over the last 18 years, she has expanded her interest on developing analytical protocols for real-time in-situ life-detection in planetary-like materials and extreme environments worldwide. Since 2009, in collaboration with the Death Valley’s National Park Service (NPS), Rosalba has been studying the distribution of life indicators in support of the Mars Science Laboratory mission, or other planned life detection missions to Mars. Over the past seven years, Rosalba has been implementing innovative laboratory experiments to simulate the Saturn’s moon Enceladus environments to support future flyby life detection missions to this icy world and other Ocean Worlds of our Solar System.
For the past eight years, Dr. Rosalba Bonaccorsi has been the experimental lead of a novel project simulating ice grain plume ejecta for Icy Moons/Ocean Worlds science at the NASA Ames Vertical Gun Range (AVGR) facility. The project helped understand past data from the Cassini flybys on Saturn's icy moon, Enceladus. Rosalba hypervelocity impact experiments involve ice target setups, biological and organic sample preparation, and contamination control to prepare for future life detection missions to the ocean worlds of our solar system.
- 2019 NASA Group Achievement Award to the Atacama Rover Astrobiology Drilling Studies (ARADS)
- 2015 NASA Group Achievement Award to the “Ames 75th Anniversary Open House Champions”.
- 2013 NASA Group Achievement Award to the “NASA Ames Family”.
- 2007 NASA Group Achievement Award to 2006 Spaceward Bound Atacama Desert Expedition.
- 2006 NASA Group Achievement Award to Mars Analog Research Technology Drilling Experiment.
Relevant Papers
Bonaccorsi, R., Willson, D., Gold, R., Adams, E., Ricca, Cornelison, C.J., Wiens, J.-P., Zheng, D., Perez, A.J. and McKay, C.P., 2024 Production of high velocity micron-sized ice particles using the NASA Ames Vertical Gun Range for application to missions to icy moons. Icarus, 421, 116203. https://doi.org/10.1016/j.icarus.2024.116203
Spry J, B Siegel, C Bakermans, D Beaty, M Bell, J Benardini, R. Bonaccorsi, S Castro-Wallace, D Coil, A Coustenis, P Doran, L Fenton, D Fidler, B Glass, S Hoffman, F Karouia, J. Levine, M Lupisella, JTorres, R Mogul, K Olsson-Francis, J Martin-Torres, R Mogul, S Ortega.-Ugalde, M Patel, D Pearce, M Race, et al (2024). Planetary protection knowledge gap closure enabling crewed mission to Mars. Astrobiology 24(3). https://home.liebertpub.com/publications/astrobiology/99
Bonaccorsi R et 8 others. (2024); Astrobiology, 24(3). Correction to: Astrobiology 2023;23(12):1303–1336. https://doi.org/10.1089/ast.2022.0133.correx
Bonaccorsi R, Glass B, Moreno-Paz, M., García-Villadangos M, Warren-Rhodes K., Parro V, Manchado J M, Wilhelm, M-B, McKay, CP (2023). In-situ Real-time Monitoring for Aseptic Drilling: Lessons Learned from the ARADS field Contamination Control Strategy and Implementation, Astrobiology, 23(12):1303-1336.
Glass BJ, Bergman D, Parro V, et al. including Bonaccorsi, R. (2023) The Atacama Rover Astrobiology Drilling Studies (ARADS) Project. (2023) Astrobiology, 23(12); doi: 10.1089/ast.2022.0126.
Bonaccorsi, R. and McKay, CP (2023) The Arid to Hyper-arid Transition in desert environments: A window into the Future of Life under a Global Warming scenario. In prep. for submission to the Int. J. of Astrobiology
Pandey, S., Clarke, J., Nema, P., Bonaccorsi, R., et 16 al. (2019) Ladakh: Diverse, High-Altitude Extreme Environments for Astrobiology Research" Int. J. of Astrobiology 19(1) https://doi.org/10.1017/S1473550419000119.
Cockell, CS et. 49 al., including Bonaccorsi, R. (2018). Subsurface scientific exploration of extraterrestrial environments (MINAR 5): analogue science, technology and education in the Boulby Mine, UK, Int. J. Astrobiol, 18, 157–182. https://doi.org/10.1017/S1473550418000186
Powers, L.S., Smith, HD., Kilungo, AP., Ellis, WR., McKay, CP. Bonaccorsi, R., Roveda, JW. (2018). In situ real-time quantification of microbial communities: Applications to cold and dry volcanic habitats, Global Ecology and Conservation, Vol 16. https://doi.org/10.1016/j.gecco.2018.e00458.
Mogul, R., Vaishampayan, P., Bashir, M., McKay, CP, Schubert, KE., Bonaccorsi, R, et. 41 others. (2017) Microbial Community and Biochemical Dynamics of Biological Soil Crusts Across the Central Mojave Desert, Front. Microbiol., 8:1974. Terrestrial Microbiology.
Willson, D., Rask, JC, George, SC., De Leon, P, Bonaccorsi, R., Blank, J, Slocombe, J, Silburn, K, Steele, H, Gargano, M., McKay, CP (2014). The performance of field scientists’ observations of early fossil life in-sim space suits Acta. Astron, 93, 193-206.
Davé, A., Thompson, S., McKay, C.P., Zacny, K., Paulsen, G., Mellerowicz, B., Glass, B.Willson, D., Bonaccorsi, R., and Rask, J. 2013. The Sample Handling System For The Mars Icebreaker Life Mission: From Dirt To Data, Astrobiology, 13(4):354-369.
Bonaccorsi, R., McKay, CP., & Chen, B. (2010). Biomass and habitability potential of clay minerals-and iron-rich environments: Testing novel analogs for Mars Science Laboratory landing sites candidates, Phil. Magazine, 90, 2309-2327.
Fairén, A.G., Davila, A.F., Lim, D., Bramall, N., Bonaccorsi, R., Zavaleta, J., Uceda, E.R., Stoker, C.R., Wierzchos, J., Amils, R., Dohm, J.M., Andersen, D. & McKay, C.P., 2010. Astrobiology through the Ages of Mars: The Study of Terrestrial Analogs to Understand the Habitability of Mars, Astrobiology. 10(8):821-843.
Stoker, CR et 31 al., including Bonaccorsi, R. (2008). The 2005 MARTE Robotic Drilling Experiment in Rio Tinto Spain: Objectives, Approach, and Results of a Simulated Mission to Search for Life in the Martian subsurface. Astrobiology, 8(5): 921-945.
Parro, V., et 10 al., including Bonaccorsi, R. 2008. SOLID2: An antibody array-based life detector instrument in a Mars Drilling Simulation Experiment (MARTE). Astrobiology, 8(5): 987-999.
Davila, AF, Fairén, AG & 8 al. w/ Bonaccorsi, R. (2008). Subsurface formation of oxidants on Mars and implications for the preservation of organic biosignatures. EPSL, 272:456-463.
Miller, D.P., Bonaccorsi, R., and Davis, K, 2008. Design and Practices for use of Automated Drilling and Sample Handling in MARTE While Minimizing Terrestrial and Cross Contamination. Astrobiology, 8(5): 947-965
Bonaccorsi, R., and Stoker, C.R. (2008). Science results from a Mars drill simulation (Río Tinto, Spain) and ground truth for remote science observations. Astrobiology, 8(5), 967-985.
Cannon, H.N., Stoker, C.RDunagan, . S.E Davis, K. Gomez-Elvira, J. Glass, B.J., Lemke, L.G. Miller, D., Bonaccorsi, R., Branson, M. Winterholler, S. and Zavaleta, J. (2007). MARTE: Technology Development and Lessons Learned from Mars Drilling Mission Simulation. Journal of Field Robotics, 24(10):877–905.
Bonaccorsi, R., T. Quaia, L.H Burckle, R.F. Anderson, R. Melis, and A. Brambati, (2007). C-14 age control of pre- and post-LGM events using N. pachyderma preserved in deep-sea sediments (Ross Sea, Antarctica). In Antarctica: A Keystone in a Changing World – Online Proceedings of the 10th International Symposium on Antarctic Earth Sciences. edited by A.K. Cooper and C.R. Raymond et al., USGS Open-File Report 2007-1047, 4 p.
Duncan, R., Tarduno, J., Scholl, D., and The Leg 197 Shipboard Scientific Party including Bonaccorsi, R. (2003). ODP Leg 197: a paleomagnetic test for motion of the Hawaiian Hotspot. JOIDES J., 29(1):2-5.
Shipboard Scientific Party, including Bonaccorsi, R. 2002. ODP, Initial Reports 197: College Station, TX (Ocean Drilling Program), p.1-92. CD online version.
Bonaccorsi, R., Finocchiaro, F., Quaia, T., and Brambati, A. 2000. From basal till to open-water sedimentation: late Pleistocene to Holocene Changes in Core ANTA95-77C2 (G. Challenger Basin - Ross Sea, Antarctica) Terra Antarctica Reports 4, 185-198.
Bonaccorsi, R., Brambati, A., Busetti, M. and Fanzutti, G.P., 2000, Relationship among X-ray lithofacies, magnetic susceptibility, P-waves velocity and bulk density in Core ANTA95-89C (Ross Sea, Antarctica). Terra Antarctica Reports 4, 241-258.
Presentation at conferences
Bonaccorsi, R., Glass, B.J, Stoker, C.R and McKay, C.P (2024) Contamination Control in Non-clean Room Settings: Lessons from the Atacama Rover Astrobiology Drilling Studies (ARADS) and Application to Ocean Worlds. Planetary Protection Panel (PPP.3), 45th COSPAR Scientific Assembly, 13 - 21 July 2024, Busan, South Korea
Bonaccorsi, R., Glass, B.J and McKay, C.P (2024) Swiss Army Tool for Safer and Faster Ocean Worlds Aseptic Sampling - Lessons from Astrobiology and Technology Field Drilling Trials. AbSciCon (Astrobiology Science Conference) May 5-10, Abstract [# 1499561].
https://agu.confex.com/agu/abscicon24/meetingapp.cgi/Paper/1499561
Glass B.J, Stoker C, Bonaccorsi R., Wilhelm, M.B (2024) Field Analog Lessons-learned in the ARADS Deployments. AbSciCon May 5-10, Abstract [# 118-02]. https://agu.confex.com/agu/abscicon24/meetingapp.cgi/Paper/1499593
Bonaccorsi R., Willson D., Gold R., Adams E., Ricca, A., Cornelison C.J., Wiens J-P., Zheng D., Perez A. J., McKay C. P. (2024). Production of High Velocity Micron-Sized Ice Particles Using the NASA Ames Vertical Gun Range for Application to Missions to Icy Moons. 55th LPSC, 55th LPSC (Lunar Planetary Science Conference), March 11-15, 2023, abstract [#3040].
Bonaccorsi, R., Willson, D., Gold, R., Ricca, A., McKay, C.P., Adams, E. (2022). Taming the Untamable: Making an Enceladuls-like plume by hypervelocity oblique impacts at the NASA Ames Vertical Gun Range, Paper #31594 COSPAR, Athens (Greece); 16-24 July.
Bonaccorsi, R. and McKay, CP (2022) Rainfall Monitoring at the Extremes: The Arid to Hyper-arid Transition and the Future of Life. Paper #318-4 318 - Astrobiology and the Future of Life: Evidence, Analogy and Prediction. AbSciCon, 15-20 May, Atlanta, GA
Bonaccorsi, R., Bishop, JL, Burton, ZFM (2020). Salty clay sites in the Mojave Desert as analogues for Mars: VNIR spectroscopy investigations of fluvio-lacustrine volcaniclastic deposits. 51st. Lunar Planet. Sci. Conf., Paper #2525.
Tiscareno, M.S., Bonaccorsi, R., Bishop, J.L., Bywaters, KF., Cody, AM., Caldwell, D.A., Tarter, J., and 13 others, (2020). A virtual REU program in Astrobiology and Planetary Science at the SETI Institute. American Geophysical Union, Fall Meeting 2020, abstract #ED035-0006.
Bonaccorsi, R., McKay, C.P., and Willson, D. (2019), 2011-2018: Engaging the Public with MarsFest Events in Death Valley National Park, Paper #407-6 AbSciCon Seattle, Washington, June 24-28.
Bonaccorsi, R., Willson, D., Gold, R., Adams, E.Y., and McKay, C.P. (2019). Small, Fast, and Cold! Enceladus Plume Analog Simulation Experiments, Paper #402-5, AbSciCon Seattle, Washington, June 24-28, 2019
Adams, E., Willson, D., Ricco, A., Gold, R.E., Bonaccorsi, R., and McKay C.P. 2018. EFun: the Plume Sampling System for Enceladus, 42nd COSPAR Scientific Assembly. 14-22 July 2018, Pasadena, California, USA, Abstract ID. B5.3-63-18.
Willson, D., Bonaccorsi, R., Gold, R., Adams, E., Ricco, A.J., and McKay, C.P. 2018. Contamination Management of Sample Collection Devices and Ocean Analogs for Life Detection on Icy World Plume Fly-through Missions. 42nd COSPAR Scientific Assembly. 14-22 July 2018, Pasadena, California, USA.
Bonaccorsi, R., Wilson, D., Davila, A., Stoker CR., McKay CP. (2017) Life Detection in Briny Environments. Planetary Science Vision 2050 Workshop #8246, LPI No. 1989.
Bonaccorsi, R, McKay, C. P. Mogul, R. Boston, P. Willson*, D., Heldmann, J., Baker, L. Cowan, D. Pandey*, S., Sharma, M., Sun, H., Blank, J.G., Stoker, C.R. Mogosanu, H., Campbell, I., Phartiyal, B., Rask, J.C. Clarke, J. and the 2006-2016 SB Teams, (2017). Spaceward Bound’s 11-Year History: To Extreme Environments on Earth and Beyond. AbSciCon, 2017.
Chiar, J., Phillips, C.B., Rudolph, A., Bonaccorsi, R., Tarter, J., Harp, G., Caldwell, D.A., DeVore, E. K., (2016) Life in the Universe - Astronomy and Planetary Science Research Experience for Undergraduates at the SETI Institute. American Geophysical Union, Fall Meeting 2016, abstract #ED51B-0799.
Bonaccorsi, R., Fairen, A., Willson, D., McKay, C.P., Mahaffy, P.R., Zent, A., 2015. Hollow Nodules gas escape sedimentary structures in Lacustrine Deposits on Earth and Gale Crater (Mars). AGU Fall Meeting, San Francisco 14-18 December 2015. Paper #PS23A-4258
Bonaccorsi, R., Baker, L., McKay, C.P., Mahaffy, P.R., Zent, A.P., Willson, D. 2015 Hollow Nodules in Lacustrine Deposits as new Proxy for Habitable Environments on Earth and Mars, Astrobiology Science Conference 2015, Paper #7719.
Bonaccorsi, R., Mckay, C.P., Baker, L., Willson, D. Zent, A. 2015 Hollow Nodules Biosignatures in Lacustrine Deposits on Earth and Mars: Pizza or Pancakes? Astrobiology Science Conference 2015 (2015), Paper #7087.
Bonaccorsi, R., Zent, A., McKay, C.P., 2014. Monitoring Surface Moisture of Crater-fill Sediment in Extreme Hydroclimatic Conditions (Ubehebe Volcanic Field, Death Valley, California). AGU Fall Meeting, San Francisco 15-19 December 2014. Paper #EP53A-3632.
Radu, H.I., Bonaccorsi, R., and Radu, C.E 2014. Erosion Effects of Liquid Water and Volatiles in a Former Lacustrine Environment - From Gale Crater to Death Valley. AGU Fall Meeting, San Francisco 15-19 December 2014. Paper#P31D-4013.
Bonaccorsi, R., Baker, L., Zent, A.P., Valdre', G, Willson, D., Friese, R., Mckay, C.P. 2014 Source- to- Sink of Crater Fill Deposits in Arid Hydroclimatic Conditions (Ubehebe Volcanic Field, Death Valley, CA). 2014. Paper No. 175-13. 2014 GSA Annual Meeting in Vancouver, British Columbia, 19–22 October 2014.
Bonaccorsi, R., Baldino, T., Jones, A.J.P. Kyriazis, S.F., Devore, E., Fuhrmann, K.K., Mckay, C.P., Willson, D., Mahaffy, P.R., and Bleacher, L.V., 2014. Marsfest Events and on Site Outreach Activities in Death Valley Natl. Park: A Journey Into A Crater 2014. Paper No. 258-8. 2014 GSA Annual Meeting in Vancouver, British Columbia, 19–22 October 2014.
Bonaccorsi, R., Jones, A.J.P., Baldino, T., Bleacher, L.V., and C.P. McKay 2014. MarsFest: an Annual Planetary Analog Event Hosted by Death Valley National Park. [abstract 2904]. 45th LPSC March 18–22, 2013, The Woodlands, Texas.
Jones, A.J.P., Bonaccorsi, R., Kyriazis, S., Baldino, T., Bleacher, L.V., and L. Coe, 2013. Mars And The Mojave: A Planetary Analog Festival In Death Valley National Park. [abstract 2846]. 44th LPSC March 18–22, 2013, The Woodlands, Texas.
Bonaccorsi, R., Friese, R., McKay, C.P., and Willson, D. 2012. Planetary Analog Research and Climate Change Monitoring in a Land of Extremes: The Ubehebe Volcanic Field (Death Valley, CA). AGU Fall Meeting, San Francisco 3-7 December 2012. Abstract #P11B-1839.
Bonaccorsi, R., Kyriazis, S., Coe, L, and McKay, C.P, 2012 Astrobiology Field Analog Research, Education, and Conservation at the Ubehebe Volcanic Field (Death Valley National Park, California): Discovery and Awareness for a possible tomorrow. AbSciCon 2012 #1132, 16-20 April, Atlanta, GA.
Bonaccorsi, R., Willson, D., McKay, C.P, Valdre', G., 2012 Astrobiology field research in a High-Fidelity Desert Analog Site: The Ubehebe Volcanic Field (Death Valley, California). Astrobiology Science Conference, AbSciCon 2012 #3102, 16-20 April, Atlanta, GA.
Bonaccorsi, R., Shirey T.B., Vimercati, L., McKay, C.P., and Chen, B., 2010. Astrobiology of Clays: Habitability potential and microRaman detection of biosignatures (LPS, ATP, and DNA) in clay minerals analogs for Mars Science Laboratory and Exomars missions. Paper IAA-S10-0203. 2nd SETI Conference, London 2010 - IAA Symposium on SEARCHING FOR LIFE SIGNATURES, 6-8 October 2010, Kavli Royal Society International Centre, London (UK).
Bonaccorsi, R. and McKay, C.P. 2009. Gram-negative Biomass in Clay Minerals Analogs: Testing Habitability Potential for the 2011 Mars Science Laboratory Mission, Eos, Vol. 90, Number 52, 29 December 2009, Fall Meet. Suppl., Abstract P43C-1444.
Bonaccorsi, R., McKay, C.P., 2009 Preservation of Biosignatures in Phyllosilicates vs. Iron-rich Environments as Mars Analogues. 9th Australian Mars Exploration Conference. 17th - 19th July 2009. Adelaide, South Australia.
Bonaccorsi, R., Stoker, C.R. & the MARTE Team. 2009.Organics-bearing phyllosilicates under hyperacidic and oxidizing conditions: preservation potential and application to mars. Oral pres. Symposium MC1: Clay Minerals in Extraterrestrial Environments. XIV International Clay Conference, Castellaneta Marina, Italy, 14-20 June 2009.
Bonaccorsi, R. and McKay, C.P. 2009. Microbial biomass in clays from arid/ hyper-arid environments: testing for habitability using Mars analogues. Oral presentation. Symposium BC1: Clays&Biomolecules: From the Origin of Life to Medical Applications. XIV International Clay Conference, Castellaneta Marina, Italy, 14-20 June 2009.
Tanaka, Z., Beer, T., McKay, C.P., Bonaccorsi, R., Gu, C., and Chen, B.. 2009. Raman Imaging for High Throughput Biomarker Field Detections. Instruments and Methods for Astrobiology and Planetary Missions XII. Edited by Hoover, R; Levin, B; Gilbert V; Rozanov, A.Y; Retherford, K.D. SPIE Proceedings, 7441: 74410I-74410I-6.
Bonaccorsi, R. and McKay, C.P. 2009. Microbial biomass in clays from arid-hyperarid regions: testing for habitability in desert mars analogs. 23rd annual Desert Symposium, California State University's Desert Studies Center, Zzyzx, CA. Oral.
Bonaccorsi, R. 2008. Organics in Phyllosilicate-rich materials from the Rio Tinto, Spain and their relevance to phyllosilicate-bearing outcrop seen on Mars. Clay Mineral Society Symposium, 8 March, New Orleans.
Bonaccorsi, R., and McKay, 2008 Total biomass and organic carbon along a N-S moisture gradient of the Atacama Region, Chile [abstract 1489]. In 39th Lunar and Planetary Science Conference Abstracts, LPI Contribution, Lunar and Planetary Institute, Houston.
Bonaccorsi, R., Stoker, C.R and The MARTE Science Team. 2008. Preservation of Organics in the Rio Tinto System: Unraveling Geochemical Patchiness in a Subsurface Mars Analog. The 5th Astrobiology Science Conference. Santa Clara, CA, April 14-17 2008.
Miller D. and Bonaccorsi, R. 2008 Lessons Learned from Microbial Contamination in MARTE Field Tests. AbSciCon 2008. Santa Clara, CA, April 14-17 2008.
Bonaccorsi, R., Stoker, C.R., and the MARTE Science Team. Organics in Aseptic Rock Samples From the Mars Analog Rio Tinto Drilling Experiment 2007 Bioastronomy2007 15-20 July 2007 San Juan (Puerto Rico).
Bonaccorsi, R., Quaia, T., Burckle, H.L. Anderson, R.F, and Brambati, A., 2007. C-14 age control of pre- and post-LGM events using N. pachyderma preserved in deep-sea sediments (Ross Sea, Antarctica). 10th International Symposium on Antarctic Earth Sciences. USGS-OFR 2007, Extended Abstract, p.1-4.
Bonaccorsi, R., Stoker, C.R., and the MARTE Science Team. Organics in Aseptic Rock Samples From the Mars Analog Rio Tinto Drilling Experiment: What lies beneath? 2007 LPSC
Bonaccorsi, R., Stoker, C.R., and the MARTE Science Team. 2006. Subsurface Organics in Aseptic Cores From the MARTE Robotic Drilling Experiment: Ground truth and Contamination Issues. AGU Fall Meeting, San Francisco 11-15 December 2006.
Glass, B., Cannon, H., Bonaccorsi, R., and Zancy, K., 2006 Mechanical Alteration and Contamination Issues in Automated Subsurface Sample Acquisition and Handling. AGU Fall Meeting, San Francisco 11-15 December 2006
Politi, R.; Marzo, G.A., Bonaccorsi, R., et al., 2006 Recognition of microfossils in rocks using Infrared and Raman Spectroscopy: a potential for Biosignatures Detection. COSPAR, 24-27July 2006-Beijing. China.
Bonaccorsi, R., Stoker, CR. and the MARTE Project Team, 2006 Subsurface Organics in Aseptic Rock Samples From the Mars Analog Rio Tinto Experiment: What Life lies Beneath? – AbSciCon-06, Washington DC, 26-30 March, 2006.
Bonaccorsi, R., and Mancinelli, R.L, 2006. A Model for the formation of Sub-Basement Fossil Soil Drilled in the North Pacific (ODP Leg 197): A Possible Novel Analog for a deep subsurface biosphere on Earth and Mars. AbSciCon-06, Washington DC, 26-30 March, 2006.
Brambati, A., Bonaccorsi, R., Quaia, T., and Busetti, M., 2003. Searching for Last Glacial Deep-Sea Polar Carbonates in the Ross Sea Continental slope and Their Relevance to Chronological Constraints. Eos Trans. AGU, 84(46), Fall Meet. Suppl., Abstract xxxxx-xx, 2003.
Bonaccorsi, R., Burckle, L., Brambati, A., Piotrowski, M.A., Quaia, T., Finocchiaro, F. and Piani, R. 2000. Multi-component analyses on Diamicton Mud Grains (GC Basin, Ross Sea, Antarctica). Suppl. to EOS, May 9, 2000. P. 269.
Chen, B., Cabrol, N., Stoker, C.R, McKay, C.P., Bonaccorsi, R., Zavaleta, J., Dunagan, S., Rodriguez-Manfredi, JA., Gomez Elvira, J, and Rul, F. 2007 Raman spectra identifications of mineral and organic constituents. _Bin_C4P3_NSTC-07-0114.pdf. NASA Science Technology Conference (NSTC2007) June 19-21 Univ. of Maryland University College.
Book Chapters
Bonaccorsi, R., Brambati, A., Burckle, L.H, and Piotrowski, A, M., 2005 Constraining subglacial settings using clay-supported IRD (Mud Grains) in Antarctic Marine Sediment: a framework for Astrobiology. NATO Science Series, I: Life and Behavioural Sciences. Perspectives in Astrobiology (Eds. R.B Hoover, A. Rozanov, R. Paepe), IOS Press, Amsterdam, The Netherlands. (366):221-232. ISBN 978-1-58603-512-9.
Bonaccorsi R. (2004) Using Stable Isotopes (C, N) to Constrain Organics in Sub-basement Fossil Soils (Ocean Drilling Program-Leg 197; N. Pacific): a Possible Example of Isolated Atmosphere-Land-Ocean Systems. IAEA International Conference on Isotopes in Environmental Studies – Aquatic Forum 25– 29 October 2004 Monte-Carlo Monaco. Paper N. IAEA-CN-118 138.
Bonaccorsi, R. and Mancinelli, R.L. 2004. The Discovery of Organics in Sub-Basement Fossil Soils Drilled in the North Pacific (ODP Leg 197): Their Model Formation and Implications for Astrobiology Research; R. Chapter VIII “Earth Analogues of Extraterrestrial Ecosystems” In: From the Miller Experiment to the Search for Life on Other Worlds Series: Cellular Origin, Life in Extreme Habitats and Astrobiology, Vol. 7 Seckbach, J.; Chela-Flores, J.; Owen, T.; Raulin, F. (Eds.) 2004 387pp ISBN: 978-1-4020-3093-2
Bonaccorsi, R., 2004 Total Organic Carbon in Red Paleosoils and Basalts from ODP Leg 197 and their potential use as suitable models for Mars soil analogues. In IAU vol. 213, BIOASTRONOMY 2002: LIFE AMONG THE STARS, ed. R. P. Norris & F. H. Stootman (San Francisco: ASP), 325-337.
Bonaccorsi, R., and Melis, R., 2001 Persistence of living planktonic foraminifera (N. pachyderma) in Antarctic sea-ice from a study of a sediment core (Ross Sea Continental Margin), pp. 255-260. In: First Steps in the origin of Life in the Universe. Chela-Flores, J., Tobias Owen, and Francois Raulin (eds). Kluwer Acad. Publ: Dordrecht.
NASA Spaceward Bound (SB) Expeditions Team member/Science Instructor/other expeditions
- Sept. 5– 25, 2019: Atacama Rover Astrobiology Drilling (ARADS) Expedition, Chile
- March 8 – 27, 2019: Atacama Rover Astrobiology Drilling (ARADS) Expedition, Chile
- October 16 – 22, 2017: Spaceward Bound (SB) Expedition MINAR 5, UK
- August 7 – 21, 2016: India & Tibetan Plateau Leh-Ladakh SB Expedition
- January 15 – 23, 2015: New Zealand SB Expedition.
- March 22 – 28, 2014: Mojave Desert (SB) Expedition (California).
- June 13 – 18, 2013: Idaho SB Expedition (USA).
- June 17 – 22, 2012: Idaho SB Expedition (USA).
- April 21 – May 2, 2012: Namib Desert SB Expedition (Namibia).
- April 2012: Mojave Desert SB Expedition
- July 9 – 20, 2011: Pilbara SB Expedition (WA).
- June 25 – 30, 2011: Idaho SB Expedition (USA).
- March 20 – 25 2011: Mojave Desert SB expedition
- April 13 – 26, 2010: Namib Desert SB Expedition (Namibia).
- March 2010: Mojave Desert SB Expedition
- May 2010: Atacama Desert SB expeditions (Chile).
- June 7 – 16, 2009: South Australian Desert SB Expedition (SA).
- March 23 – 27, 2009: Mojave Desert SB expedition
- March 20 – April 14, 2008: Atacama Desert SB expeditions (Chile).
- March 25 – 30, 2007: Mojave Desert SB expedition
- June 19 – July 1, 2006: Atacama Desert SB expedition (Chile).
- May – June 2006: Rio Tinto NASA MARTE expeditions (Spain).
- March 2006: Mojave Desert SB expedition
- 2005: Rio Tinto MARTE expeditions (Spain).
- January 2005: Shetland Islands and Antarctic Peninsula expedition onboard RV Explorer.
- September 2004: Rio Tinto NASA MARTE expeditions (Spain).
Education activity in Death Valley National Park
- 2021 – present: Field Education events lead Death Valley Natural History Association (DVNHA) https://dvnha.org/program-events/exploring-space-from-death-valley/
- 2013 – 2014: DVNHA Road Scholar Program Field Instructor
- 2020 – present: lead Death Valley Death Valley Dark-Sky-Festival events https://www.nps.gov/deva/planyourvisit/death-valley-dark-sky-festival-field-talks.htm
- 2011 – 2019: Co-funder and Organizing Committee for the annual “Mars & Mojave Festival” & “MarsFest” events 2013, Death Valley Natl. Park https://www.seti.org/event/join-us-marsfest-february-23-25-2018
1. Multi-component analysis of Mars-like surface materials
(a) Iron oxyhydroxides-rich, Sulfates-rich and clay-rich sediments and rocks from modern volcanic terrains (Death Valley Natl. Park, California).
(b) Clay-rich, Carbonates and Sulfate-rich deposits from the Atacama Desert (Chile).
(c) Gravel-supported soil (alluvial) from the Atacama Desert
(d) Intra-dune clay-rich deposits (Namib Desert Sand Sea).
(e) Modern soil from Hawaii (weathering product of basalts).
(f) Soils from the Shetland Islands (Antarctica).
(g) Soils from the Antarctic Dry Valleys and coastal Antarctica. See examples in Figure 1 and Figure 2.
2. High-resolution stratigraphy of subsurface materials (~300 drill cores)
(a) Miocene-Pliocene marine/lacustrine facies (Eastern Mediterranean Sea).
(b) Clay-supported Ice Rafted Debris (IRD) (Ross Sea, Antarctica).
(c) K/T Boundary North Pacific biogenic deep sediments (siliceous and calcareous microfossils (ODP Leg 197, Emperor Seamounts)
(d) ~ 1km sub-basement lava flows and fossil soils beds (ODP Leg 197, Emperor Seamounts, North Pacific).
(e) ~ 100 m deep Volcanogenic massive sulphide ore deposits and near-surface
(6 m) ironstone/clays deposits (Rio Tinto, Spain, MARTE Project).
3. Long-term water cycle monitoring in Death Valley Natl.
Park 2009-present: Managing data loggers of soil/air microclimate conditions (moisture, temperature and rainfall). Over 130,000 hrs of instrumental data logged in the last 3 years including ~2500 hrs of direct field observation at study site (Ubehebe Volcanic Field).
• Terrestrial/geological applications: Intra-crater fine-grained sediments store information on decadal cycles of wet and dry years. Individual storm-generated layers inform on intensity, amount and duration of rainfall storms.
• Planetary applications: Miocene to modern Mars-like fluvio-lacustrine deposits in cratered terrains as natural laboratory to understand on how (1) amount of intra-crater liquid and solid transport; (2) deposition of clay-rich fill sediment, and frequency and life cycle of freshwater ephemeral lakes relate to (a) production, (b) concentration, (c) preservation, and (c) detection of biosignatures.
4. MicroRaman Spectroscopy (soil, minerals, sediments, clean surfaces)
• General applications: Non-destructive characterization of terrestrial environments, identification of mineral particles vs. biological organic components (microbes and their cellular constituents) in soil, sediments, and rocks (See example in Figure 1).
• Planetary Protection: Detection of putative microbes in mineral dust-coated spacecraft surfaces to prevent cross-contamination of planetary environments.
5. Optical identification of mineral and biological particles in sediments and rocks.
8-year experience in analysis of smear slides (SS) samples. SS enables fast and cheap qualitative/semi quantitative compositional analysis of fine-grained particles via optical microscopy.
• Applications: Marine geochemistry (low T); study of provenance, diagenesis, and transport processes in low vs. high-energy environments; sample characterization and selection; material characterization to resolve ambiguities of microRaman Spectroscopy (Figure 1) and XRD analysis (Figures 1.2).
6. Use of non culture-based biological assays e.g., LAL (Lymulus Amebocite Lysate) and ATP (adenosine triphosphate) Luminometry.
Modifying and implement extraction protocols for rocks and sediments. Assessing total and metabolically active biomass in geological environments.
Terrestrial and Planetary Applications: (a) Detection of viable microbial components (bioburden) on spacecrafts and geological surfaces (Planetary Protection issue); b) Discrimination of living vs. fossil life in geological matrices; (c) testing for habitability potential as planetary analog materials, and (d) use the results to best inform on how and where to search for life on a planetary surfaces with analogue geology.
7. Bulk organic geochemistry. “Flash” combustion CN Elemental Analysis.
17+ experience in quantification of total carbon, organic carbon, and nitrogen in geological and biological materials.
• Terrestrial/geological applications: carbon/nitrogen cycles; soil productivity; soil ecology; palaeoenvironmental/palaeoclimatic assessment; provenance-to-sink studies.
• Industrial applications: Materials & food characterization; evaluation of compost maturity and source components (Quality Control).
• Planetary Applications: Discrimination of organic/inorganic carbon pools in planetary samples; preservation potential in mineral rich environments;. Identification/mitigation of false positive/negative data.
Examples
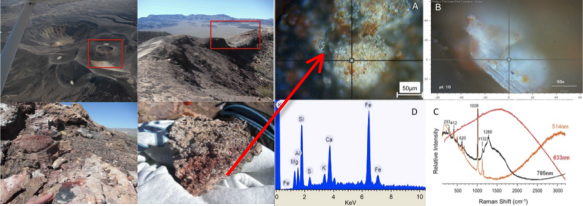
Figure 1. From Macro to Micro: Left-hand side: Aerial view of a cratered volcanic terrain with the Little Hebe Crater framed. Right-hand side: Distinct microRaman spectra signatures of gypsum-cemented rocks from the rim (b) simultaneously showing mineralogical and microbiological spectral signatures of gypsum at 1008, 620, 493 and 211cm–1 (at 785 nm) and hematite at 293, 412, 498 and 660 cm–1. Cyanobacterial colonies band at 1280 cm–1 is assigned to the scytonemin pigment (at 785 nm). The assignments are supported by compositional EDX spectra (d), and optical observations (a, b 20 X microscope objective). Modified from Bonaccorsi et al., 2010.
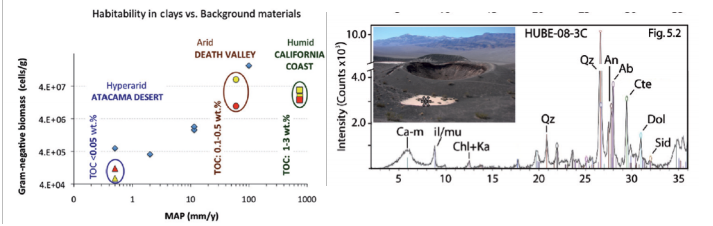
Figure 2A (left). Biomass concentrations of phyllosilicate- (light-toned symbols) versus Fe-oxide-rich samples (dark-toned) along the rainfall gradient. Left to right: samples from (1) the Atacama Desert (triangles), (2) Death Valley, Calif. (dots), and Pescadero Beach, Calif. (squares) sites. Total Organic Carbon content information (TOC, wt%).
Figure 2B (right). X-Ray Diffractogram (background subtracted raw data) for samples from the Little Hebe Crater. Sample analyzed for Lypopolisaccaride (lipid A) biomarker in Fig. 2A. Illite/smectites mixed layer (I-S); illite (ill); chlorite (chl); kaolinite (ka); Camontmorillonite (ca-m); muscovite (mu); quartz (Qz); albite (Ab); anortite (An); calcite (ctc); dolomite (Dol); and siderite (Sid).